When I dropped out of school for biomedical engineering 5 years ago, I didn’t think I’d ever get a paper published in an academic journal. The desire for having a place to put ideas forward in a way they may be judged and improved is part of what prompted me to start this Substack.
That said, I recently submitted my first scientific article for peer review. Why did I take the time to do this? This is an expensive, time consuming and bureaucratic process. Generally speaking, I am for the decentralization of science movement. I think the barriers to entry for contributing to the domain of public knowledge should be more open but that we should still have mechanisms in place for rigorous assessment of ideas and information.
I undertook the process for the same reason I attended a Gordon Research Conference last May on my own dime. A GRC conference is a setting for scientists to come together and present pre-publication research to their peers. GRC manages multiple conferences per year, each centered around a very specific domain. When I caught wind of an inaugural gathering coming up for Systemic Processes, Omics Approaches and Biomarkers in Aging, a voice inside my head told me I had to go.
I ended up writing a few people in the space who I admired, including a few past guests we’d had on The Vance Crowe Podcast. I did not have the support of an institution to attend, meaning that if I was to go for the weeklong event, I’d have to pay my own way, totaling thousands of dollars after tickets and travel. I wanted to see if the people I looked up to would think it was worth the expense for someone in my position—an independent with interests in these ideas but not actively working in the space; financially comfortable, but not so well off it was an easy expense to swallow.
Of the 5 people I wrote, 3 told me to hang back, and 2 told me to jump on the opportunity.
The anecdote that you don't know what's right for you until someone else tells you what they think struck me here. This is the idea that once you hear someone else’s opinion, you suddenly realize what you truly want.
I wanted to go. I wanted to be there. I wanted to because I had spent the 4 years prior since dropping out continuing to allocate time and attention towards teaching myself the subjects I could not afford to in school.
I attended, and wrote about the experience in a previous post here.
The reason this relates to having recently submitted a paper to a journal is this: I had do it to see if I could, to keep moving forward.
At the conference, I left my bubble and learned I could hold conversations with the top researchers in my field of interest. I returned home after the event with a renewed sense of momentum that has stuck with me for the last year of continued time and investment into developing my ideas.
The publication I’ve put forward is the culmination of that effort. It is also the top of a new local optima. I’ve combed the landscape far and wide to craft my argument for a new path forward in the form of this literature review, but now I once again need to venture into a new type of unknown to continue making progress.
As a part of this effort, I will be presenting these ideas live at the Foresight Institute’s Longevity Frontiers Workshop in San Francisco on April 17 - 18, and in Montenegro at Zuzalu’s Longevity Industry Talks on May 13 - 14.
Below is an outline of these talks, starting with the
State of the art
I will be exploring the concept of bioelectricity and discussing its potential to significantly impact our current approaches to tackling aging. I feel strongly that this research has the potential to determine optimal strategies for efficient, precise, and translational rejuvination.
Before I do that though, I want to set the stage by talking about the state of the field on the industry side, and what domain is getting most of the attention currently.
In 2022, $5.2 billion dollars were invested into companies tackling longevity across 130 deals. Of that money invested, 49% went towards ventures pursuing an approach that involved cellular reprogramming in one way or another.1 For context, this is nearly five times the percentage allocated to the next highest domain of discovery platforms which revived 11% of funding. This is largely due to one major investment in a single company, but even so, Longevity.Technology's report explains:
While cellular reprogramming’s top spot is solely due to Altos Labs, it does warrant a separate mention. First of all, even without Altos Labs, cellular reprogramming would still be in the Top 10 over the last 5 years, and secondly, the fact that it is concentrated in one company does not diminish the impact on the field. Since the groundbreaking work by Takahashi and Yamanaka in 2006 which first demonstrated that age-related cellular changes can be reversed, the field of cellular reprogramming has gained a great deal of traction. The revolutionary possibilities that cellular reprogramming holds, if it can be mastered, would have immense impact on health span and even lifespan.
Key terminology here is ‘if it can be mastered’.
The potential benefits of achieving successful cellular reprogramming in vivo are clear. This process indicates it is possible to remove age related damage, rejuvenate aged cells, delay age related disease and extend healthy lifespan.
However, there are a handful of major hurdles yet to be overcome before translating these results to a full organism including:
Risk of tumorigenicity
Need to develop cell-specific delivery methods
Limited understanding of underlying mechanisms
Limited ability to control differentiation
High cost and complexity of the procedure
Cellular reprogramming is really promising. There's good reason it captured nearly 50% of last year's capital invested into longevity companies. But, we have a long way to go before we see this research translate to whole organism rejuvenation therapies.
Enter,
Bioelectricity: A new hope
So now that I've talked about where most of the field is paying attention to, I want to switch gears to highlight bioelectricity as it may be implicated in aging.
For the last 3 and a half years, I have filled the role of executive producer for The Vance Crowe Podcast. On our show, we interview people who spot patterns in business, culture and science that others don't—or at least haven't yet.
In November of 2021 at the height of my personal disillusionment with cellular reprogramming, I reached out to Dr. Michael Levin at Tufts University to talk about the work his lab was doing to uncover novel regenerative medicine therapies. Michael spoke with Vance about what he referred to as the bioelectric code, and how he believed this was the ideal information processing medium through which to achieve more effective whole organism intervention strategies.
Below is one of the most striking examples of this from Levin's lab. This is a planaria. Planaria are highly regenerative species and are also effectively immortal which makes them an interesting case study in the context of aging. The planaria pictured here is unique because as you can see, it has 2 heads.
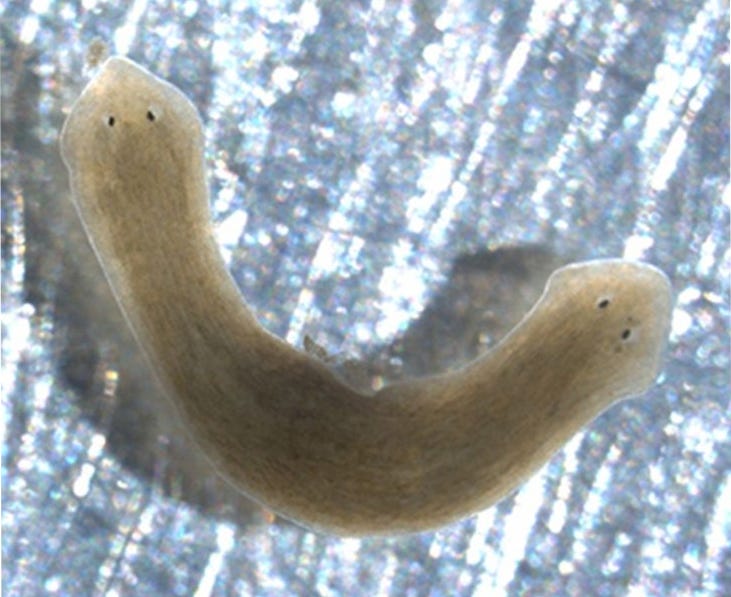
This two-headed planaria was not created by altering its genome. Instead, its bioelectric state was manipulated after being cut in half. What's even more remarkable is that this two-headed characteristic persisted as the organisms continued to reproduce, showing that bioelectric interventions alone can have lasting effects on an organism's physical traits. I’ll talk more about how this was achieved below.
First, a
Bioelectricity primer
An important concept to understand regarding bioelectricity is membrane voltage. This is the electrical potential difference between a cell's internal cytoplasm and it's extracellular environment. Changes in membrane voltage are part of what causes neurons to fire and carry signals in the brain, however on slower time scales, all cells work similarly. A cell will have resting or ‘steady state’ membrane potential that when polarized or hyperpolarized to a given voltage from the transport of certain molecules, will cause other downstream transcriptional processes to occur.
Membrane voltage is implicated in a number of aging mechanisms, many of which I go into in great detail in my above mentioned paper. Here, I will focus on 2, starting with
Mitotic division
Mitotic division—or mitosis— is a process that occurs in eukaryotic organisms where two genetically identical daughter cells are formed from a single parent cell. This process is crucial for growth, development, and repair of tissues in multicellular organisms. Mitotic disfunction is a well established hallmark of aging.
In a series of groundbreaking studies in the late 1960s, Clerance Cone was able to induce a reversible mitotic block by altering the intracellular ionic concentration of cells, and also showed that sustained depolarization could induce DNA synthesis and mitosis in mature cells2. Since Cone's initial work, other researchers have expanded upon these findings, examining the role of specific ion channels and molecular mechanisms that regulate membrane voltage in various cell types. Although these initial studies were groundbreaking, they were limited by the available technology and methods at the time. Subsequent research has built upon Cone's findings using more advanced techniques.
The illustration below demonstrates the importance of special gate-like structures, called voltage-gated ion channels, in helping cells progress from one phase of their life cycle to another. These channels regulate the movement of charged particles such as potassium (K+), sodium (Na+), and calcium (Ca2+) in and out of the cell.
When the cell is preparing to move from the resting phase (G0/G1) to the DNA synthesis phase (S-phase), voltage-gated potassium channels open, allowing potassium ions to flow out of the cell. This movement of positive charges from inside to outside the cell restores the cell's electrical balance, a process called repolarization, which is essential for the cell to continue its life cycle.
When the cell enters the S-phase and gets ready for cell division, the electrical balance changes again. This is due to the opening of sodium and/or calcium channels, which allow these ions to flow into the cell. The influx of these ions causes a shift in the cell's electrical balance, known as depolarization, which is necessary for the cell to divide and create two new cells.
Once the cell division is complete, the electrical balance is reestablished through repolarization in the G0/G1 phases, and the cycle continues. The precise control of these voltage-gated ion channels ensures the cell progresses through its life cycle in an orderly and efficient manner.
As organisms age, the finely-tuned regulation of voltage-gated ion channels and the cell cycle becomes disrupted. Age-related changes in the cell leads to alterations in the balance and movement of ions across cell membranes. These changes impact the proper opening and closing of ion channels, resulting in an imbalance of the cell's electrical state.
Consequently, this affects the progression of the cell cycle, leading to a decline in the efficiency of cell division, DNA repair, and overall cell function. In some cases, these age-related dysfunctions contribute to the development of age-related diseases, including neurodegenerative disorders and certain cancers. Understanding the mechanisms behind these changes and developing potential interventions to counteract them is I think one key to promoting healthy aging and combating age-related diseases.
The second key is understanding bioelectricity as it pertains to
Epigenetic modifications
Epigenetic modifications are changes that occur within cells, which influence gene expression without altering the DNA sequence itself. These modifications act like molecular switches that can turn genes on or off, or fine-tune their activity levels. The most common types of epigenetic changes include DNA methylation, where molecules called methyl groups attach to DNA, and histone modifications, which involve chemical changes to proteins called histones that help package and organize DNA within the cell.
Below is a high level breakdown of how a tissue wide voltage gradient can lead to specific changes in gene expression in individual cells. Intercellular signaling mechanisms lead to second messenger cascades that regulate epigenetic modifications and cell behavior at the single cell level. These signals may be altered in aging cells, contributing to age-related changes in gene expression or other cellular processes.
Now with this context, we can return to the incredible
Case studies
The following figure delves deeper into the process of creating a two-headed planarian. In typical planarians, there is a gradient of membrane voltage along their body, with the head-forming region being the most positively charged and the tail-forming region the least positively charged. Researchers manipulated this gradient using two treatments: one involving SCH-mediated H,K-ATPase inhibition, which led to an increased negative charge, and another using ivermectin, which resulted in an increased positive charge. When the amputated regions were hyperpolarized, they developed into either a tail or a headless planarian. In contrast, when they were depolarized, they formed a head, ultimately leading to the two-headed planarian phenotype.
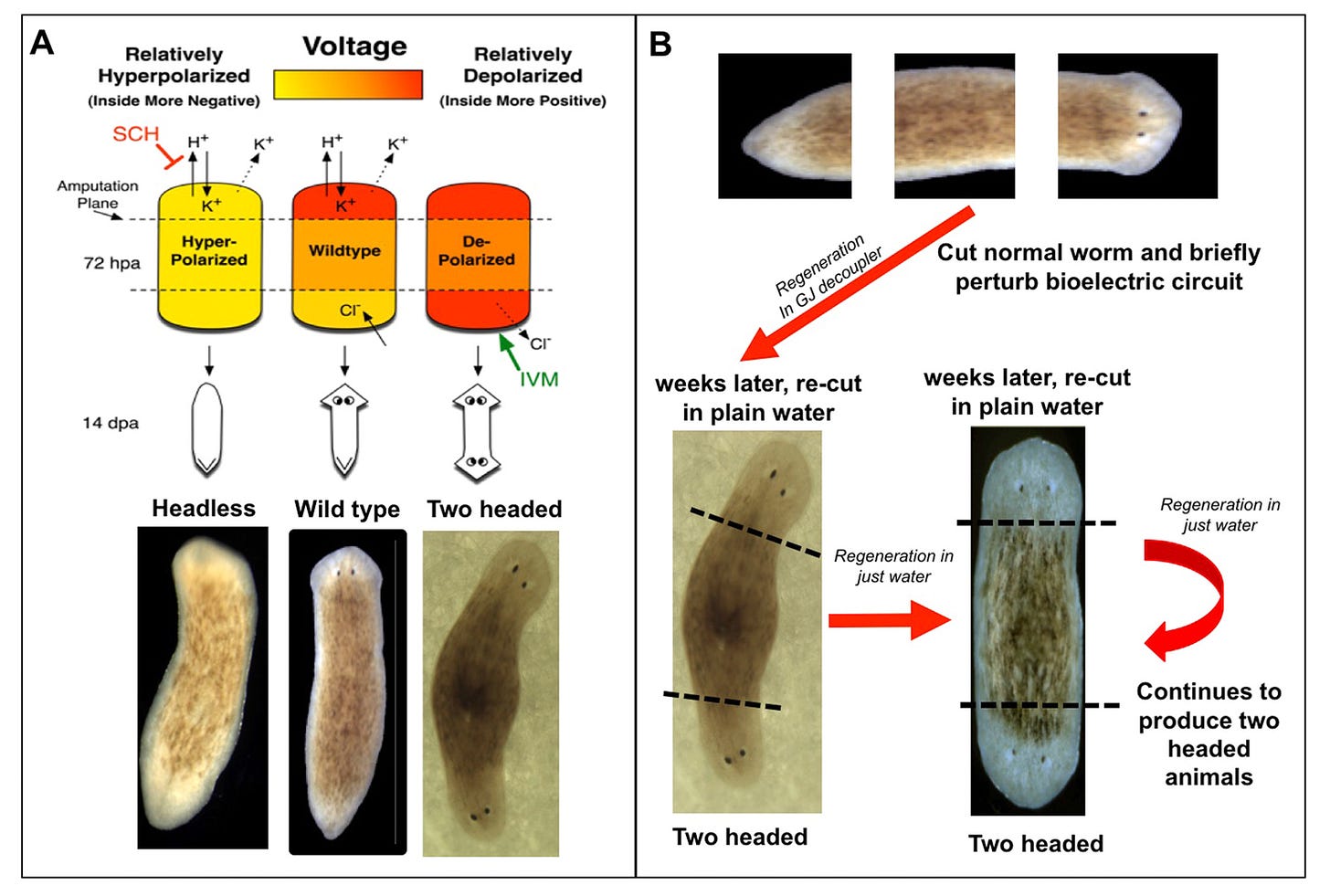
Another incredible example of bioelectric intervention from the Levin lab is that they've been able to achieve limb regeneration in post-metamorphic froglets where it does not normally occur. After only 24 hours of exposure, a small molecule cocktail designed to specifically alter the bioelectric state of an amputated area triggers growth of an entire limb.3
This example shows that a straightforward signal can set off a chain of events that lead to well-organized and properly scaled changes in the shape and structure of an organism. These changes are fine-tuned and adapted to the organism's needs and location within its body.
Exploiting such endogenous “top-down” controls may be a powerful strategy not only for regenerative medicine, but also
In the context of aging
I believe these control mechanisms have significant potential to enhance the effectiveness and precision of partial reprogramming and reveal entirely new approaches for rejuvenating whole organisms. Another advantage of researching these types of interventions is the ability to achieve whole organism results without requiring the knowledge to micromanage complex processes in specific cell types.
This field of research is no longer on the fringes. A few months ago, Levin and his colleague David Kaplan's lab spinoff, Morphoseuticals, raised $8 million to explore this work for improving amputation stump health, limb regeneration, organogenesis, and channelopathies4. Investors are willing to support these new therapeutic approaches, but there's still a long way to go in terms of funding to reach the level of attention this promising area deserves, especially concerning aging.
So, who am I to share this information? I'm not incentivized by money or prestige; my focus is on what I believe to be the most effective approach to confront an inevitable challenge that affects us all.5
It’s time to build.6
-Benjamin Anderson
Contact me sovereignly on—
Urbit: ~padlyn-sogrum
Nostr: benjamin@buildtall.com
Podcast appearances since my last post:
Shaun Newman Podcast: Archipelagos, Books and Dreams
JackSquared Podcast: Art, Gall and Morali
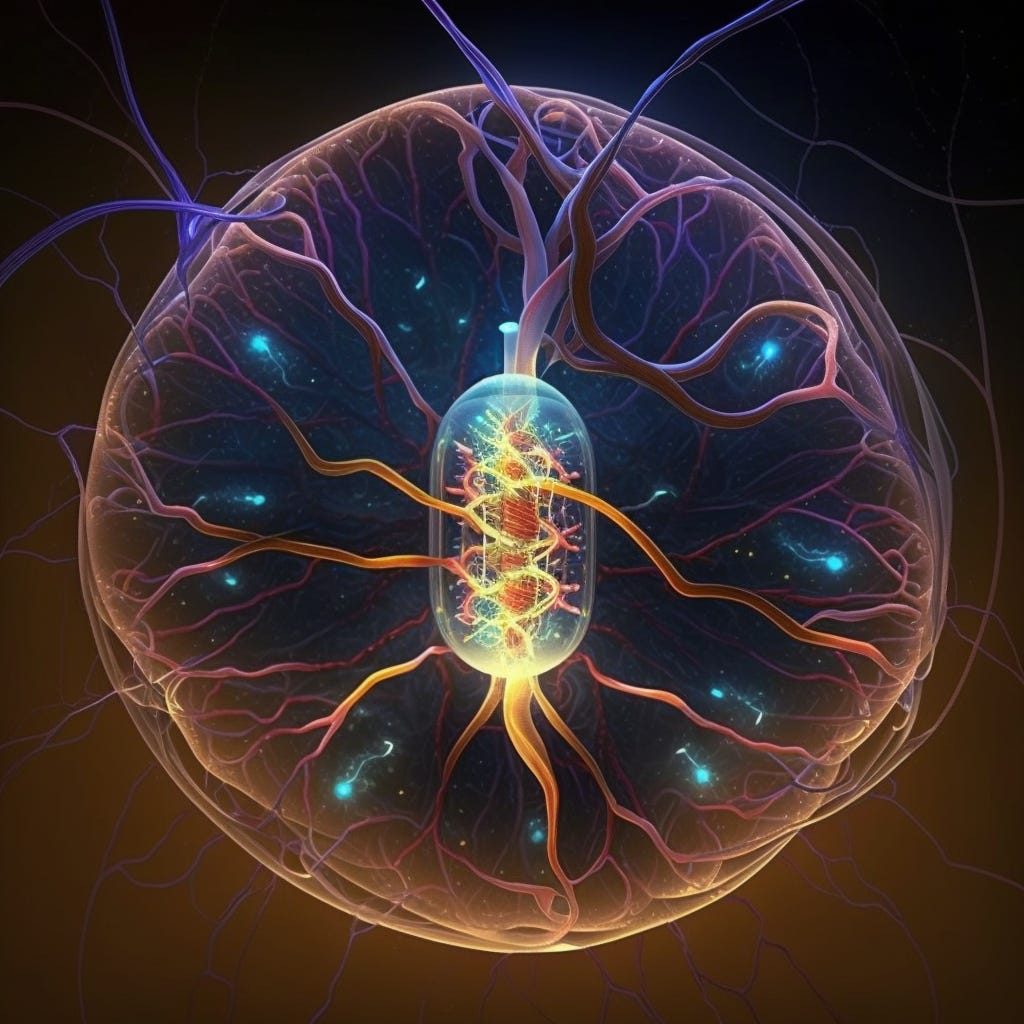
https://longevity.technology/news/global-longevity-investment-hit-5-2-billion-in-2022/
Cone CD Jr, Tongier M Jr. Control of somatic cell mitosis by simulated changes in the transmembrane potential level. Oncology. 1971;25:168-82.
McLaughlin KA, Levin M. Bioelectric signaling in regeneration: Mechanisms of ionic controls of growth and form. Dev Biol. 2018 Jan 15;433(2):177-189. doi: 10.1016/j.ydbio.2017.08.032. Epub 2017 Dec 25. PMID: 29291972; PMCID: PMC5753428.
Read the press release.
Had to quote ‘im: Alex Trapp